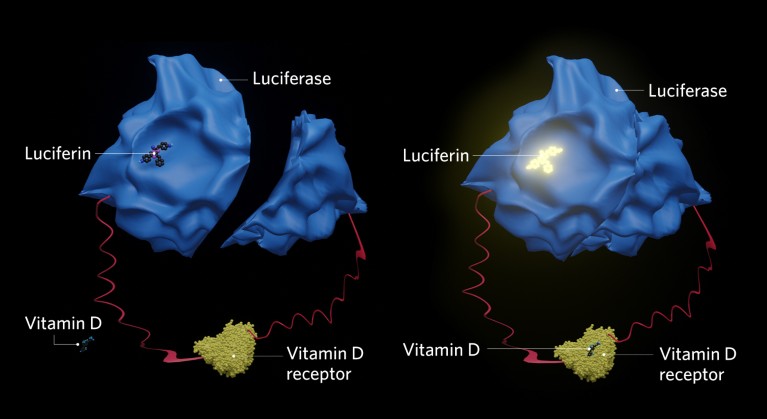
Schematic for a vitamin D biosensor based on a split-luciferase system. When a vitamin D receptor (yellow) comes into contact with a vitamin D molecule (dark blue), the two parts of the luciferase enzyme (light blue) are brought together, causing the luciferin (dark blue molecule on top of luciferase) to luminesce.
Vitamin D is one of the many essential nutrients required by the body to stay healthy. In addition to its role in bone development and maintenance, vitamin D is important for the normal functioning of the nervous system and immune system.
Over the past 30 years, research has revealed links between serum levels of this vitamin and a range of diseases including osteoporosis, obesity, metabolic syndrome, type 2 diabetes, rheumatoid arthritis, inflammatory bowel disease, respiratory bacterial infections, cancers, and viral infections, such as those caused by HIV and the SARS-CoV-2 virus.
Despite the importance of vitamin D for overall health, it is estimated that about one billion people worldwide have a deficiency, usually defined as having serum levels of 25-hydroxyvitamin D3 (25(OH)D3) — the major circulating form of vitamin D — below 20 nanograms per millilitre (ng/ml).
Sun exposure
In Japan, a recent study of 5,518 healthy people aged between 20 and 90, found that nearly 80% were deficient in vitamin D, with an overall average of 15.5 ng/ml1.
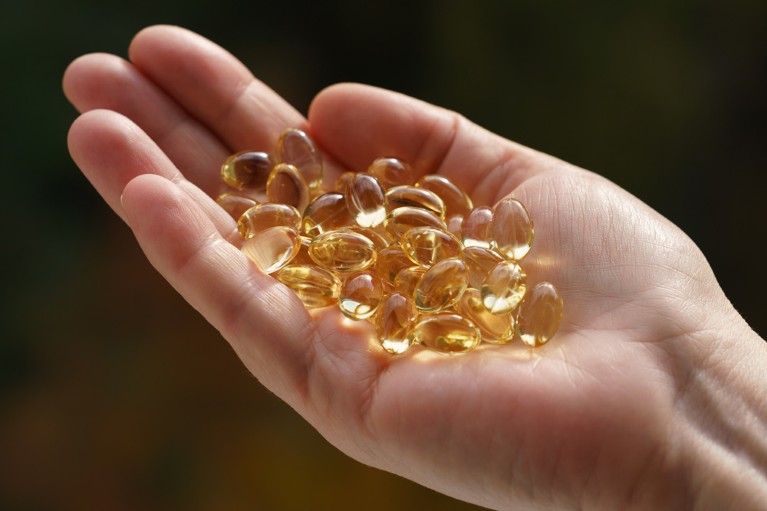
Some people take supplements to overcome their vitamin D deficiency.Credit: Yury Karamanenko/iStock/Getty
A deficiency can result from inadequate dietary intake, although very few foods naturally contain vitamin D. Deficiency is more commonly linked to insufficient sunlight exposure, since most vitamin D is produced from 7-dehydrocholesterol in skin by ultraviolet B (UVB) irradiation
Frequent cloud cover, smoggy environments, indoor lifestyles, using too much sunscreen and having naturally high levels of melanin, diminish the skin’s ability to produce vitamin D. Deficiencies often go unnoticed, with only those that are extreme and prolonged manifesting as rickets in children and osteomalacia (or soft bone disease) in adults.
“Unlike blood glucose, fasting blood glucose, and hemoglobin A1c levels — which are related to prevention and treatment of diabetes and easily measured — quantifying vitamin D levels is not as simple,” says Takuya Kushioka, a researcher at the FANCL Research Institute, the research branch of cosmetics company, FANCL Corporation, based in Yokohama, Japan.
Kushioka believes vitamin D levels should be measured during annual medical check-ups. “Despite its association to various diseases, even I do not know my own vitamin D level because I have never had the opportunity to measure it,” he says.
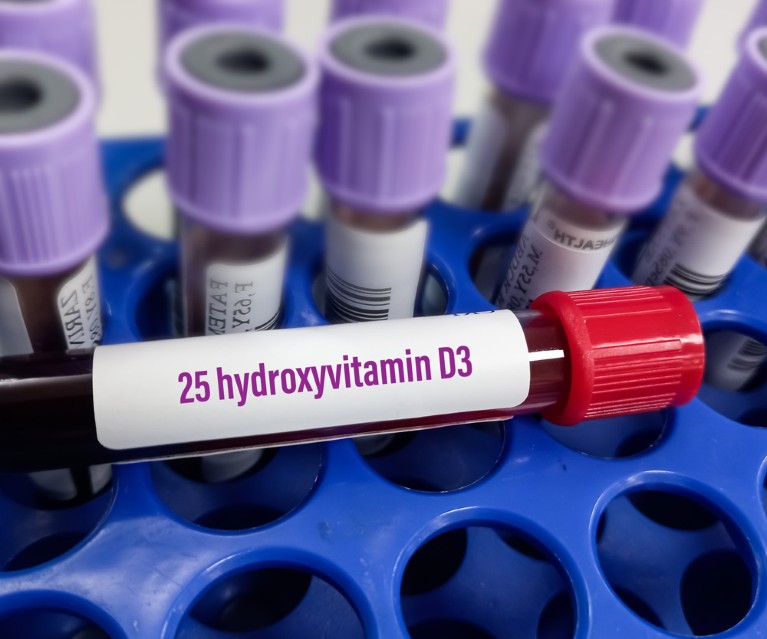
The major circulating form of vitamin D is 25-hydroxyvitamin D3.Credit: Babul Hosen/Shutterstock
Current methods to measure vitamin D levels require a blood sample and rely on automated immunoassays or high-pressure liquid chromatography mass spectrometry to detect 25-hydroxyvitamin D3 or the active form of Vitamin D3 (1α,25(OH)2D3). Because of the need to draw blood — and the cost and time required to perform the test — it is usually only offered to people who are at high risk of vitamin D deficiency, such as those with gastrointestinal problems who have problems absorbing vitamins and nutrients. It is also sometimes offered to those who are taking specific medications, have osteoporosis or get limited sun exposure.
In addition, both methods have their sensitivity and selectivity challenges. More than 99% of 25(OH)D3 in blood is bound to the vitamin D binding protein, albumin and other carriers, which can interfere with signal generation and detection. Furthermore, despite standardization efforts, there is still considerable variability between assays, complicating accurate measurement of Vitamin D status.
Vitamin D biosensor
Kushioka, in collaboration with Hiroki Mano and Toshiyuki Sakaki, at Toyama Prefectural University, (TPU) in Imizu, Japan, has been developing a sensitive vitamin D biosensor that estimates serum levels from urine. “Our system can predict vitamin D serum levels in less than 1 ml of urine,” says Sakaki, who is a professor emeritus of pharmaceutical engineering at TPU and an expert on vitamin D metabolism.
The sensor detects the interaction between vitamin D metabolites and the ligand-binding domain (LBD) of the vitamin D receptor. It is based on a split-luciferase system, whereby the light-producing enzyme is split in two and attached to the interacting proteins of interest, so that when the interaction occurs, the two parts are brought together to form active luciferase.
“By using a nanoluciferase system bound to the LBD of the vitamin D receptor and to the co-activator amino-acid sequence ‘LXXLL’, we hope to have drastically improved the sensitivity and speed of detection,” Sakaki explains.
In early versions of the sensor, the LBD of the vitamin D receptor was fused to both domains of firefly luciferase (LucC-LBD-LucN). In cells2 and cell-free systems3, Sakaki and colleagues showed that the sensor could detect a conformational change upon binding of vitamin D receptor ligands in a dose and time-dependent manner.
“Although we were using the system to screen for candidate therapeutic compounds with vitamin D receptor affinity, we soon realized that it could be used for the diagnostic measurement of vitamin D status,” says Mano.
The researchers were able to increase the sensitivity of the sensor 10-fold by modifying the system to detect the interaction between LBD and co-activating proteins upon Vitamin D receptor agonist binding4. This was achieved by fusing the luciferase domains to LBD and to the ‘LXXLL’ amino-acid sequence found on co-activators. The sensitivity of detection was further improved by using a nanoluciferase, which is smaller, more stable and brighter than firefly luciferase5. Therefore, this detection system is able to accurately quantify trace amounts of vitamin D.
The value of urine
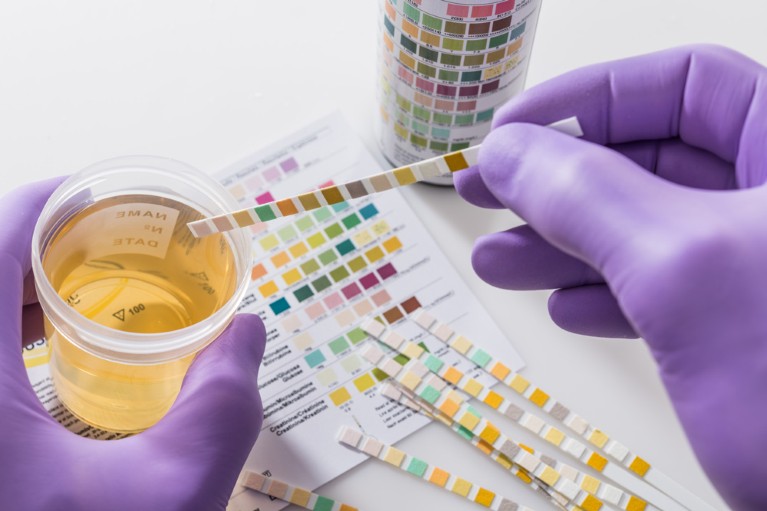
Researchers are working on a biosensor to detect 25-hydroxyvitamin D3 in urine.Credit: Lothar Drechsel/iStock/Getty
There is great interest in developing assays to measure trace levels of vitamin D, and other nutrients, in urine as a cost-effective way to improve diet and health.
“In some countries, at-home vitamin D deficiency tests are available, but they require a finger prick blood sample. To determine vitamin D sufficiency status in a more convenient and less painful way, we turned our attention to urine,” says Kushioka.
When the NLucVDR assay system was used to measure vitamin D metabolites in the blood and urine of 23 individuals, the researchers showed a correlation with the levels measured using an immunoassay or mass spectrometry6. They found that the NLucVDR was particularly sensitive to the 25(OH)D3 levels in urine.
“We are still investigating the association between urinary and blood vitamin D levels, the affinity of NLucVDR to different vitamin D metabolites, and testing the system in a larger population,” Kushioka says.
His team is also exploring the best ways to provide nutrition and health guidance, based on the test results, to prospective users. “Our aim is to contribute to disease prevention and healthy longevity by assessing vitamin D sufficiency levels, cheaply, quickly and non-invasively,” he concludes.